熒光激光雷達(dá)光譜油品污染的識(shí)別
瀏覽次數(shù):1008 發(fā)布日期:2024-6-14
來(lái)源:本站 僅供參考,謝絕轉(zhuǎn)載,否則責(zé)任自負(fù)
Oil pollution discrimination by an inelastic hyperspectral Scheimpflug lidar system
FEI GAO, JINGWEI LI, HONGZE LIN, AND SAILING HE*
Centre for Optical and Electromagnetic Research, Zhejiang Provincial Key Laboratory for Sensing
Technologies, Zhejiang University, Hangzhou 310058, China
*sailing@zju.edu.cn
Abstract: An inelastic hyperspectral Scheimpflug lidar system is developed for rangeresolved oil pollution detection and discrimination. A theory of system parametric design is built for aquatic circumstances, and laser-induced fluorescence spectra with an excitation wavelength of 446 nm are employed to detect oil pollution. Seven kinds of typical oil samples are tested and well distinguished using the principal component analysis (PCA) and linear discriminant analysis (LDA) methods. It has been shown that blue laser diodes (LD) have great potential for oil pollution detection, and our system could be further utilized for more applications in both marine and terrestrial environments.
© 2017 Optical Society of America
OCIS codes: (280.0280) Remote sensing and sensors; (010.3640) Lidar; (300.2530) Fluorescence, laser-induced.
References and links
1. M. D. C. Martín, N. V. Yarovenko, C. P. Gómez, J. L. L. Soto, and J. M. T. Palenzuela, “Oil pollution detection using spectral fluorescent signatures (SFS),” Environ. Earth Sci. 73, 2909–2915 (2015).
2. X.-l. Li, Y.-h. Chen, J. Li, J. Jiang, Z. Ni, and Z.-s. Liu, “Time-resolved fluorescence spectroscopy of oil spill detected by ocean lidar,” in Optical Measurement Technology and Instrumentation, (International Society for Optics and Photonics, 2016), 101550Q.
3. M. Fingas and C. Brown, “Review of oil spill remote sensing,” Mar. Pollut. Bull. 83(1), 9–23 (2014).
4. C. E. Brown and M. F. Fingas, “Review of the development of laser fluorosensors for oil spill application,” Mar. Pollut. Bull. 47(9-12), 477–484 (2003).
5. T. Hengstermann and R. Reuter, “Lidar fluorosensing of mineral oil spills on the sea surface,” Appl. Opt. 29(22), 3218–3227 (1990).
6. S. D. Christesen, C. N. Merrow, M. S. DeSha, A. Wong, M. W. Wilson, and J. C. Butler, “Ultraviolet fluorescence LIDAR detection of bioaerosols,” in SPIE's International Symposium on Optical Engineering and Photonics in Aerospace Sensing, (International Society for Optics and Photonics, 1994), 228–237.
7. S. Svanberg, “Fluorescence lidar monitoring of vegetation status,” Phys. Scripta 1995, 79 (1995).
8. G. Cecchi, L. Pantani, V. Raimondi, L. Tomaselli, G. Lamenti, P. Tiano, and R. Chiari, “Fluorescence lidar technique for the remote sensing of stone monuments,” J. Cult. Herit. 1, 29–36 (2000).
9. C. Brown, “Laser fluorosensors,” Oil Spill Sci. Technol, 171–184 (2011).
10. M. Brydegaard, A. Gebru, and S. Svanberg, “Super Resolution Laser Radar with Blinking Atmospheric Particles----Application to Interacting Flying Insects,” Prog. Electromag. Res. 147, 141–151 (2014).
11. L. Mei and M. Brydegaard, “Atmospheric aerosol monitoring by an elastic Scheimpflug lidar system,” Opt. Express 23(24), A1613–A1628 (2015).
12. L. Mei and M. Brydegaard, “Continuous‐wave differential absorption lidar,” Laser Photonics Rev. 9, 629–636 (2015).
13. E. Malmqvist, S. Jansson, S. Török, and M. Brydegaard, “Effective parameterization of laser radar observations of atmospheric fauna,” IEEE J. Sel. Top. Quantum Electron. 22, 327–334 (2016).
14. G. Zhao, M. Ljungholm, E. Malmqvist, G. Bianco, L. A. Hansson, S. Svanberg, and M. Brydegaard, “Inelastic hyperspectral lidar for profiling aquatic ecosystems,” Laser Photonics Rev. 10, 807–813 (2016).
15. M. Starzak and M. Mathlouthi, “Cluster composition of liquid water derived from laser-Raman spectra and molecular simulation data,” Food Chem. 82, 3–22 (2003).
16. L. Mei, P. Lundin, M. Brydegaard, S. Gong, D. Tang, G. Somesfalean, S. He, and S. Svanberg, “Tea classification and quality assessment using laser-induced fluorescence and chemometric evaluation,” Appl. Opt. 51(7), 803–811 (2012).
17. I. T. Jolliffe, “Principal Component Analysis and Factor Analysis,” in Principal component analysis (Springer, 1986), pp. 115–128.
18. S. Wold, K. Esbensen, and P. Geladi, “Principal component analysis,” Chemometr. Intell. Lab. 2, 37–52 (1987).
1. Introduction
Oil pollution is a serious environmental problem, as wind and waves can scatter an oil spill over a wide area of sea within just a few hours [1]. It often occurs during oil transportation or exploration processes, which seriously affects the hydrological environment and biological survival [2]. Laser fluorosensors are useful instruments and they use the phenomenon that aromatic compounds in mineral oils may absorb ultraviolet excitation light and become electronically excited. This excitation is then rapidly removed through the process of fluorescence emission, primarily in the visible region of the spectrum [3]. Furthermore, laserinduced fluorescence (LIF) emissions of naturally-occurring substances, such as chlorophyll, occur at quite different wavelengths than mineral oils [4]. As different types of oil exhibit characteristic variability of their spectral distributions, it is possible to differentiate among various classes of oil [5].
Remote sensing, which is often combined with laser-induced fluorescence technology,
plays an important role in the detection of bioaerosols [6], monitoring of vegetation status [7], investigation of stone monuments [8] and oil pollution response [2]. Most laser fluorosensors used for oil spill detection employ a pulsed laser operating in the ultraviolet region of 308- 355 nm [9]. However, the high cost and complexity of conventional pulsed lidar systems hinder their usability to researchers [10,11]. In recent years, continuous-wave laser diodes with high-power and low-cost have been developed and widely used in Scheimpflug lidar systems based on the Scheimpflug principle, which describes the relationship between image planes and object planes when they are not parallel [10–13]. Since the Scheimpflug systems were initially developed for the purpose of remote modulation spectroscopy [10], they can only detect elastic signals instead of inelastic signals, such as fluorescence or Raman signals.
In 2016, an inelastic hyperspectral lidar was realized by combining Scheimpflug lidar and
hyperspectral push broom imaging techniques in Zhao’s work, which mainly focused on small organisms in water, such as phytoplankton and zooplankton [14]. However, the system design for range measurement is approximate. In our work, the theory of system parametric design is established for the first time and is adapted for aquatic applications. To our best knowledge, it is the first time that 446 nm is employed as the excitation wavelength in a laserinduced fluorescence system for range-resolved oil pollution detection, with a compromise of weak water absorption, availability to high-power LD and reasonable fluorescence excitation efficiency. Seven kinds of typical oil samples are measured and distinguished using the principal component analysis (PCA) and linear discriminant analysis (LDA) methods. Our experimental results show that not only can ultraviolet light be used as the excitation wavelength, but also that blue LDs have great potential for oil pollution discrimination.
2. Principles and method
According to the Scheimpflug principle, when the lens and object planes are not parallel, the image plane will intersect both the lens and object planes. Theoretically, an infinite focus depth can be achieved despite using a large optical aperture.
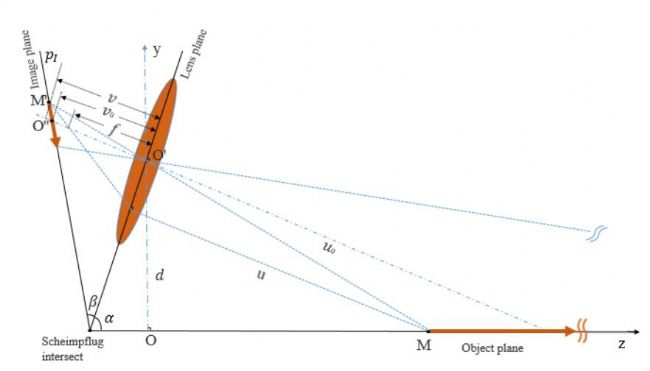
Fig. 1. Scheimpflug principle: the image plane intersects both the lens and object planes when the object plane is not parallel to the lens plane. Oʹ and Oʹʹ are the origins of the lens and image planes, respectively; pI - the pixel position of the image sensor on the image plane, d -the distance to the lens from the object plane, α - the tilt angle of the lens plane to the object plane, β - the tilt angle of the image plane to the lens plane. M is the object point and Mʹ is the image point accordingly, which satisfies the lens equation, i.e.,
Fig. 2. Scheimpflug principle applied to the underwater environment. (a) The light path which indicates that refraction of the laser beam must be taken into consideration. (b) The relationship between pixel number and distance with optical parameters: d = 0.306 m, f = 55 mm, α = 84°.